A Researcher Proposes A Model To Explain How Enzyme-substrate
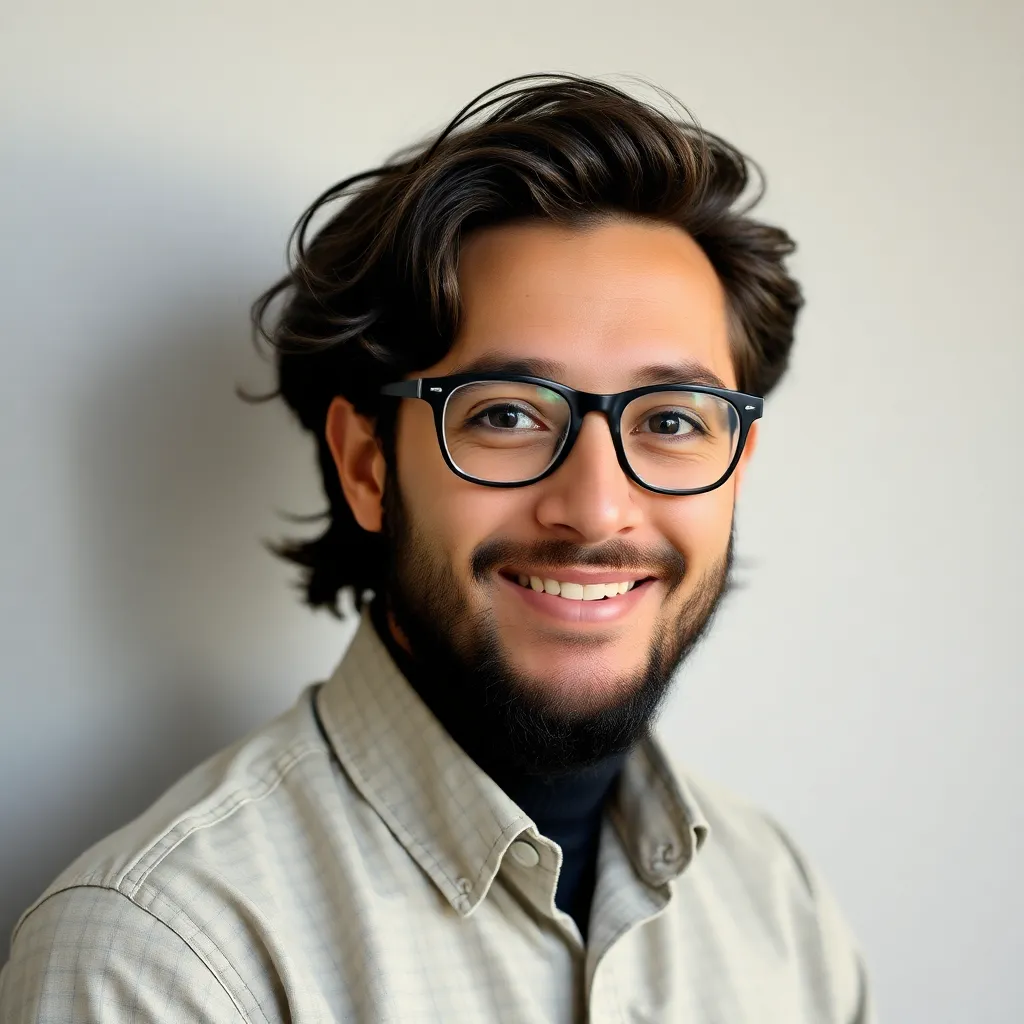
Holbox
Apr 15, 2025 · 6 min read
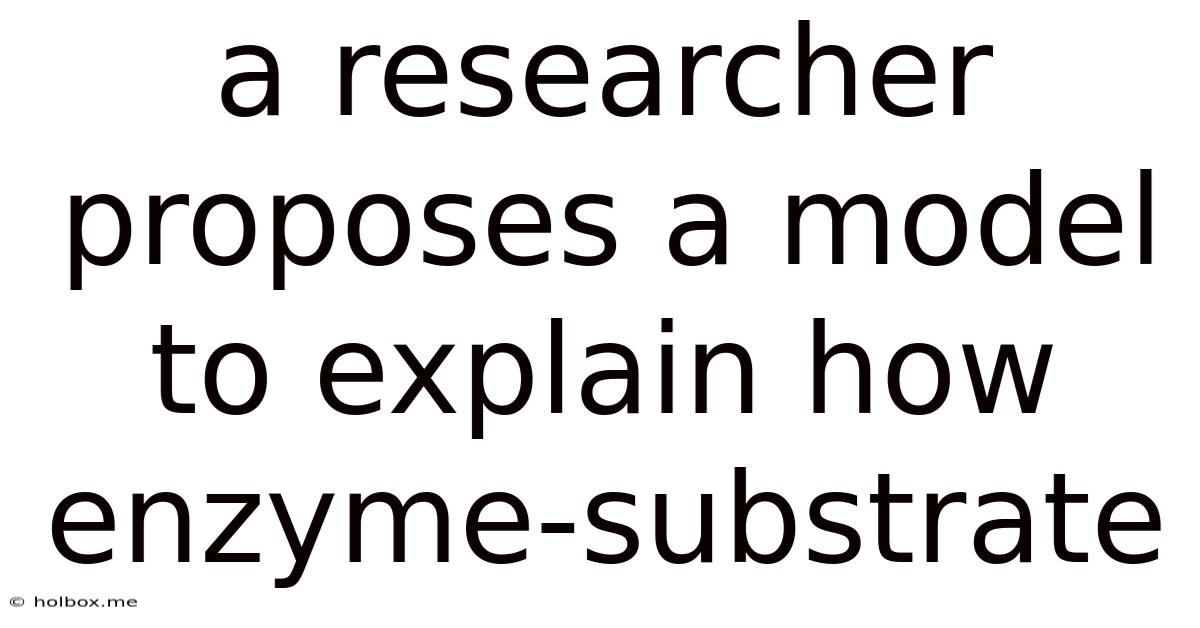
Table of Contents
- A Researcher Proposes A Model To Explain How Enzyme-substrate
- Table of Contents
- A Novel Model Explaining Enzyme-Substrate Interactions: Bridging the Gap Between Theory and Experiment
- The Challenges of Existing Models: A Critical Overview
- The Proposed Novel Model: A Dynamic and Holistic Perspective
- 1. Dynamic Conformational Landscape: Beyond Static Structures
- 2. Solvent-Mediated Interactions: The Importance of Water
- 3. Network of Interactions: Beyond Simple Binding
- 4. Transition State Stabilization: A Multifaceted Approach
- Implications and Applications of the Model
- Future Directions and Research
- Conclusion
- Latest Posts
- Latest Posts
- Related Post
A Novel Model Explaining Enzyme-Substrate Interactions: Bridging the Gap Between Theory and Experiment
Enzymes, the biological catalysts of life, orchestrate countless biochemical reactions with remarkable efficiency and specificity. Understanding the intricate dance between an enzyme and its substrate—the molecule upon which the enzyme acts—is paramount to comprehending life's fundamental processes. While existing models offer valuable insights, they often fall short in explaining the nuances of enzyme-substrate interactions, particularly the complexities of conformational changes and the role of solvent dynamics. This article explores a recently proposed model that attempts to bridge this gap, providing a more holistic and dynamic perspective on enzyme catalysis.
The Challenges of Existing Models: A Critical Overview
Traditional models of enzyme-substrate interactions, such as the lock-and-key model and the induced-fit model, provide a foundational understanding. The lock-and-key model, proposed by Emil Fischer in 1894, posits a rigid enzyme structure with a perfectly complementary active site for the substrate. While conceptually simple, this model fails to account for the flexibility observed in many enzymes and the conformational changes that occur during catalysis.
The induced-fit model, proposed by Daniel Koshland in 1958, offers an improvement by acknowledging the enzyme's conformational flexibility. This model suggests that the enzyme's active site undergoes a conformational change upon substrate binding, optimizing the interaction and facilitating catalysis. However, even the induced-fit model has limitations. It often overlooks the crucial role of solvent molecules, the intricate network of hydrogen bonds and electrostatic interactions, and the dynamic nature of the enzyme-substrate complex.
Furthermore, many existing models struggle to account for:
- The entropic cost of binding: Substrate binding often involves a loss of entropy, which can be unfavorable. Models need to explain how enzymes overcome this energetic barrier.
- The role of water molecules: Water molecules play a critical role in enzyme catalysis, influencing both the structure and dynamics of the enzyme-substrate complex. Their effects are often neglected in simplistic models.
- The influence of conformational fluctuations: Enzymes are not static entities; they undergo constant conformational fluctuations that influence substrate binding and catalytic efficiency.
- The mechanism of transition state stabilization: Enzymes enhance reaction rates by stabilizing the high-energy transition state, but the precise mechanisms of this stabilization are often not fully elucidated.
The Proposed Novel Model: A Dynamic and Holistic Perspective
The proposed model builds upon the foundations laid by previous models but incorporates several crucial advancements to address the limitations mentioned above. This innovative approach integrates the following key elements:
1. Dynamic Conformational Landscape: Beyond Static Structures
This model emphasizes the dynamic nature of enzymes, treating them not as rigid structures but as entities constantly exploring a complex conformational landscape. The enzyme's conformational flexibility is not merely a passive response to substrate binding but an active participant in catalysis. The model incorporates computational simulations, such as molecular dynamics (MD) simulations, to map this conformational landscape and identify key conformational states crucial for catalysis.
2. Solvent-Mediated Interactions: The Importance of Water
The role of water molecules is explicitly integrated into the model. Water molecules are not treated as passive bystanders but as active participants that influence enzyme-substrate interactions. The model accounts for the specific interactions between water molecules and the enzyme and substrate, including hydrogen bonding and hydrophobic effects. This consideration significantly impacts the energetic landscape of the enzyme-substrate interaction and explains the role of water in stabilizing the transition state.
3. Network of Interactions: Beyond Simple Binding
The model moves beyond simply considering the interaction between the enzyme's active site and the substrate. Instead, it recognizes the interconnected network of interactions within the enzyme and between the enzyme and its environment. This network encompasses a vast array of non-covalent interactions, including hydrogen bonds, electrostatic interactions, van der Waals forces, and hydrophobic interactions. The interplay of these interactions is crucial in directing the enzyme towards catalytically productive conformations.
4. Transition State Stabilization: A Multifaceted Approach
This model delves deeper into the mechanism of transition state stabilization, acknowledging that it is a multifaceted process involving various interactions within the network described above. The model highlights how the enzyme actively participates in desolvating the substrate and orienting it correctly for reaction. This active process enhances the binding affinity for the transition state, significantly lowering the activation energy and accelerating the reaction rate.
Implications and Applications of the Model
This new model provides a more comprehensive and nuanced understanding of enzyme-substrate interactions, offering several significant implications:
- Drug design: By providing a more accurate representation of enzyme-substrate interactions, the model can aid in the design of more potent and specific inhibitors. This is particularly relevant in the development of drugs targeting disease-related enzymes.
- Enzyme engineering: Understanding the dynamic conformational landscape and the crucial roles of solvent molecules and network interactions allows for rational enzyme engineering to improve catalytic efficiency and specificity.
- Fundamental biological understanding: The model enhances our fundamental understanding of enzyme catalysis, addressing long-standing questions and stimulating further research.
- Predictive modeling: Integrating experimental data with computational techniques allows for the creation of predictive models that can simulate enzyme-substrate interactions and predict catalytic activity under varying conditions.
Future Directions and Research
While this model represents a significant advancement, further research is necessary to refine and expand its capabilities:
- Higher resolution experimental data: Advanced experimental techniques, such as cryo-electron microscopy and time-resolved spectroscopy, can provide higher-resolution data to validate and refine the model's predictions.
- Integration of more sophisticated computational methods: Utilizing advanced computational methods, such as enhanced sampling techniques and quantum mechanical calculations, can improve the accuracy of simulations and provide deeper insights into the mechanisms of catalysis.
- Application to a wider range of enzymes: The model's applicability should be tested on a wider range of enzymes, encompassing diverse reaction mechanisms and structural classes.
- Incorporating allosteric regulation: Future iterations of the model should aim to integrate allosteric regulation, where the enzyme's activity is modulated by binding at sites distant from the active site.
Conclusion
The proposed model offers a significant leap forward in understanding enzyme-substrate interactions by explicitly incorporating the dynamic nature of enzymes, the crucial role of solvent molecules, and the interconnected network of interactions influencing catalysis. This holistic perspective allows for a more accurate description of enzyme function and offers significant implications for drug design, enzyme engineering, and fundamental biological research. Continued research and development of this model promise further advancements in our understanding of these intricate biological processes and will pave the way for innovative applications in biotechnology and medicine. The journey to unraveling the complexities of enzyme catalysis is an ongoing one, and this new model serves as a critical stepping stone on that path.
Latest Posts
Latest Posts
-
Company B Loses 1575 For Every Employee
Apr 28, 2025
-
Development Through The Lifespan 7th Edition
Apr 28, 2025
-
Common Allocation Bases For Factory Overhead Costs Are
Apr 28, 2025
-
An Evolutionary Adaptation Suits An Organism To Its Blank
Apr 28, 2025
-
What Is The Specific Heat Of Silver
Apr 28, 2025
Related Post
Thank you for visiting our website which covers about A Researcher Proposes A Model To Explain How Enzyme-substrate . We hope the information provided has been useful to you. Feel free to contact us if you have any questions or need further assistance. See you next time and don't miss to bookmark.