A Particle Level Diagram Of A Metallic Element
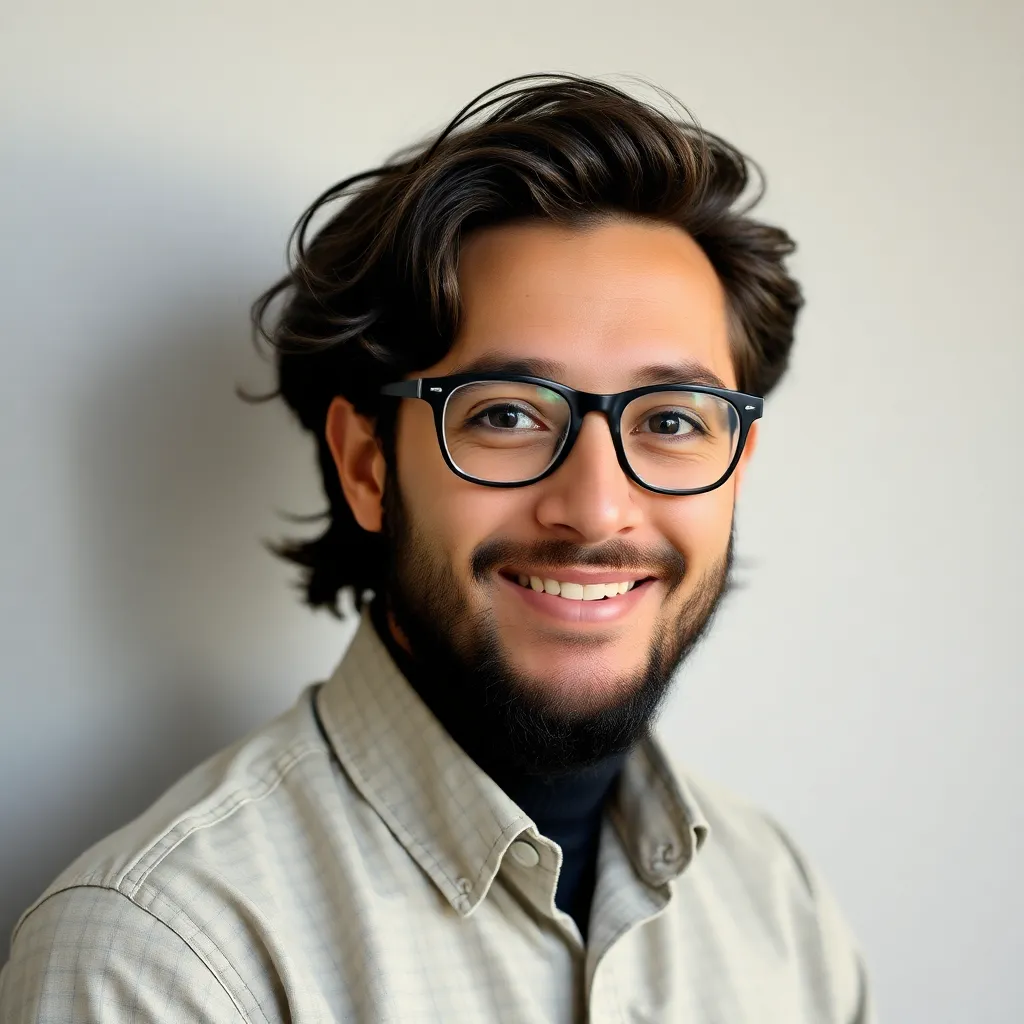
Holbox
May 12, 2025 · 6 min read
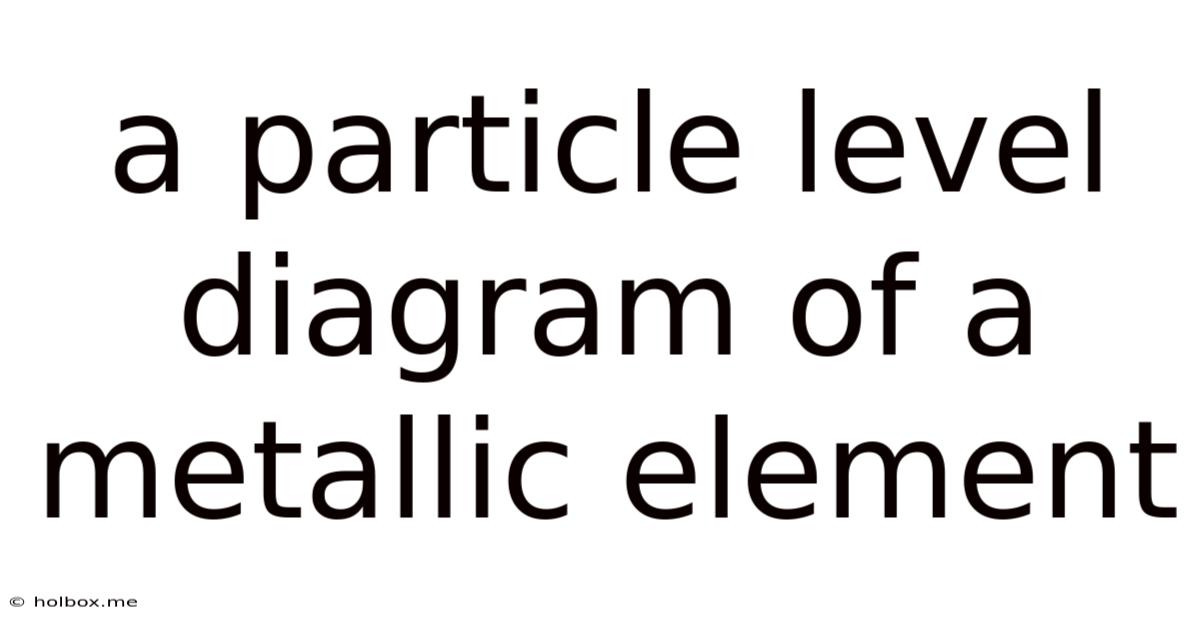
Table of Contents
- A Particle Level Diagram Of A Metallic Element
- Table of Contents
- A Particle-Level Diagram of a Metallic Element: Delving into the Atomic Structure and Properties
- The Atomic Structure of Metals: A Sea of Electrons
- The Metallic Lattice: A Regular Arrangement of Ions
- Delocalized Electrons: The Glue that Holds it Together
- Properties of Metals: A Direct Result of their Structure
- Electrical Conductivity: The Free Flow of Electrons
- Thermal Conductivity: Efficient Heat Transfer
- Malleability and Ductility: The Ability to be Shaped
- Luster: The Shine of Metals
- Density: Closely Packed Atoms
- Comparing Metallic Bonding to Other Types of Bonding
- Metallic Bonding vs. Ionic Bonding
- Metallic Bonding vs. Covalent Bonding
- Advanced Concepts and Applications
- Alloys: Modifying Properties Through Mixing
- Defects in the Metallic Lattice: Impact on Properties
- Nanomaterials: Exploring Novel Properties at the Nanoscale
- Conclusion: The Importance of Understanding Metallic Structure
- Latest Posts
- Related Post
A Particle-Level Diagram of a Metallic Element: Delving into the Atomic Structure and Properties
Understanding the behavior of metals requires a deep dive into their atomic structure. This article will explore the particle-level diagram of a metallic element, explaining the arrangement of atoms, electrons, and the forces that govern their interactions. We'll examine how this unique structure gives rise to the characteristic properties of metals, such as conductivity, malleability, and ductility.
The Atomic Structure of Metals: A Sea of Electrons
Unlike ionic or covalent compounds, metals possess a unique arrangement at the atomic level. Instead of discrete molecules, metallic elements are characterized by a sea of delocalized electrons. This "sea" surrounds a lattice of positively charged metal ions, creating a strong, yet flexible structure.
The Metallic Lattice: A Regular Arrangement of Ions
Imagine a highly organized, three-dimensional arrangement of positive metal ions. This is the metallic lattice, a repeating pattern that extends throughout the entire metal sample. The specific arrangement varies depending on the metal; common structures include body-centered cubic (BCC), face-centered cubic (FCC), and hexagonal close-packed (HCP).
- Body-centered cubic (BCC): Atoms are located at the corners of a cube, with an additional atom in the center. Examples include iron (Fe), chromium (Cr), and tungsten (W).
- Face-centered cubic (FCC): Atoms are located at the corners of a cube, with additional atoms at the center of each face. Examples include aluminum (Al), copper (Cu), and gold (Au).
- Hexagonal close-packed (HCP): Atoms are arranged in a hexagonal pattern, with layers stacked in an ABAB sequence. Examples include magnesium (Mg), zinc (Zn), and titanium (Ti).
These structures are incredibly important because they dictate many of the metal's physical properties. The tightly packed nature of the atoms leads to high density, while the regular arrangement contributes to the ability of metals to deform without fracturing (malleability and ductility).
Delocalized Electrons: The Glue that Holds it Together
The key to understanding metallic bonding lies in the delocalized electrons. These are valence electrons – the outermost electrons in an atom – that are not associated with any particular atom but rather are free to move throughout the entire metallic lattice. They are not bound to individual atoms, hence the term "delocalized."
This "sea" of delocalized electrons acts as a kind of "glue," holding the positive metal ions together through electrostatic attraction. The strong electrostatic forces between the positive ions and the negative electron sea are responsible for the strength and high melting points of many metals.
Visualizing the Electron Sea: A Simplified Model
Imagine a grid representing the metallic lattice, with positive metal ions positioned at the grid points. Surrounding these ions is a cloud or "sea" of negatively charged electrons. These electrons are not stationary; they are constantly moving and interacting with the positive ions, creating a dynamic, cohesive structure.
Properties of Metals: A Direct Result of their Structure
The unique structure of metals, with its metallic lattice and sea of delocalized electrons, directly accounts for their characteristic properties.
Electrical Conductivity: The Free Flow of Electrons
The most striking property of metals is their excellent electrical conductivity. The delocalized electrons are free to move under the influence of an electric field. When a voltage is applied, these electrons flow readily, creating an electric current. This ease of electron movement explains why metals are excellent conductors of electricity.
Thermal Conductivity: Efficient Heat Transfer
Metals are also excellent conductors of heat. The delocalized electrons, constantly in motion, readily transfer kinetic energy from one part of the metal to another. This efficient energy transfer explains why metals heat up and cool down quickly.
Malleability and Ductility: The Ability to be Shaped
Metals are known for their malleability (the ability to be hammered into sheets) and ductility (the ability to be drawn into wires). These properties are a direct result of the metallic lattice structure. When a metal is subjected to stress, the layers of atoms can slide past one another without breaking the metallic bonds. This is because the delocalized electrons are not associated with specific atoms and can adjust to the shifting arrangement.
Luster: The Shine of Metals
The metallic luster, or shiny appearance, is also linked to the interactions between light and the delocalized electrons. The electrons absorb and re-emit light, giving metals their characteristic shine. The specific color of the luster depends on the metal and the energy levels of its electrons.
Density: Closely Packed Atoms
The density of a metal is generally high due to the close packing of atoms in the metallic lattice. This compact arrangement results in a large number of atoms within a given volume.
Comparing Metallic Bonding to Other Types of Bonding
It's helpful to contrast metallic bonding with other types of chemical bonding:
Metallic Bonding vs. Ionic Bonding
In ionic bonding, electrons are transferred from one atom to another, creating positively and negatively charged ions that are held together by electrostatic attraction. This results in brittle, high-melting point compounds. Metallic bonding, on the other hand, involves a sea of delocalized electrons that bind the positive ions, leading to malleable and ductile materials.
Metallic Bonding vs. Covalent Bonding
Covalent bonding involves the sharing of electrons between atoms, forming discrete molecules. This typically results in low melting points and poor conductivity. Metallic bonding, with its delocalized electrons, creates a continuous network of atoms, leading to high conductivity and malleability.
Advanced Concepts and Applications
The understanding of the particle-level diagram of metals has profound implications for materials science and engineering.
Alloys: Modifying Properties Through Mixing
Alloys are mixtures of two or more metals, or a metal and a non-metal. By carefully selecting the components and their proportions, engineers can tailor the properties of the resulting alloy to meet specific needs. For example, adding carbon to iron creates steel, which is stronger and harder than pure iron. The addition of other elements further modifies properties like corrosion resistance and ductility.
Defects in the Metallic Lattice: Impact on Properties
Real metallic crystals are not perfectly ordered; they contain various defects, including vacancies (missing atoms), interstitial atoms (atoms squeezed into spaces between lattice sites), and dislocations (irregularities in the arrangement of atoms). These defects significantly affect the mechanical properties of metals, influencing strength, ductility, and creep resistance. Understanding and controlling these defects is crucial for optimizing the performance of metallic materials.
Nanomaterials: Exploring Novel Properties at the Nanoscale
The study of nanomaterials, materials with at least one dimension smaller than 100 nanometers, has opened up exciting new possibilities. At this scale, the properties of metals can deviate significantly from bulk behavior, leading to enhanced catalytic activity, increased strength, and other novel properties. Research into nanoscale metal structures is revolutionizing various fields, including medicine, electronics, and energy.
Conclusion: The Importance of Understanding Metallic Structure
The particle-level diagram of a metallic element reveals a complex and fascinating structure that explains many of the properties we associate with metals. The regular arrangement of positive ions within the metallic lattice, surrounded by a sea of delocalized electrons, results in excellent conductivity, malleability, ductility, and other characteristic properties. Understanding this structure is crucial for developing new materials, improving existing ones, and advancing various technological fields. Further research into the intricacies of metallic bonding and the impact of defects and nanoscale structures continues to unlock new possibilities and shape the future of materials science.
Latest Posts
Related Post
Thank you for visiting our website which covers about A Particle Level Diagram Of A Metallic Element . We hope the information provided has been useful to you. Feel free to contact us if you have any questions or need further assistance. See you next time and don't miss to bookmark.